As part of my PhD, I’m operating a dilution fridge 24/7. Recently, I found myself explaining to lot of colleagues or friends how it works, so I think a blog post about this may be quite useful.
Good electron transport measurements necessitate low temperature measurements for several reasons:
- quantum effects do not persist at high temperatures;
- the Fermi edge is sharp at low temperatures;
- lattice vibrations (phonons) are much weaker at low temperatures, inducing low electron-phonon interactions.
Ultra low temperatures
To reach and maintain low temperatures, liquid helium inside cryostat have been the mainstream technique. However, it is difficult and expensive to achieve temperatures significantly below 1K only by pumping ⁴He to low pressures. To get to lower temperatures, H. London proposed in 1951 an idea of the ³He/⁴He dilution refrigerator, that has been realised ten years later at Leiden University by H. London, G.R. Clarke and E. Mendoza, and quickly improved by BS. Neganov in Dubna and H.E. Hall at the University of Manchester⁽¹⁾. The mechanism consists in mixing two stable isotopes of helium, that cools down when it separates in two phases.
The phase diagram of ³He/⁴He is shown below:
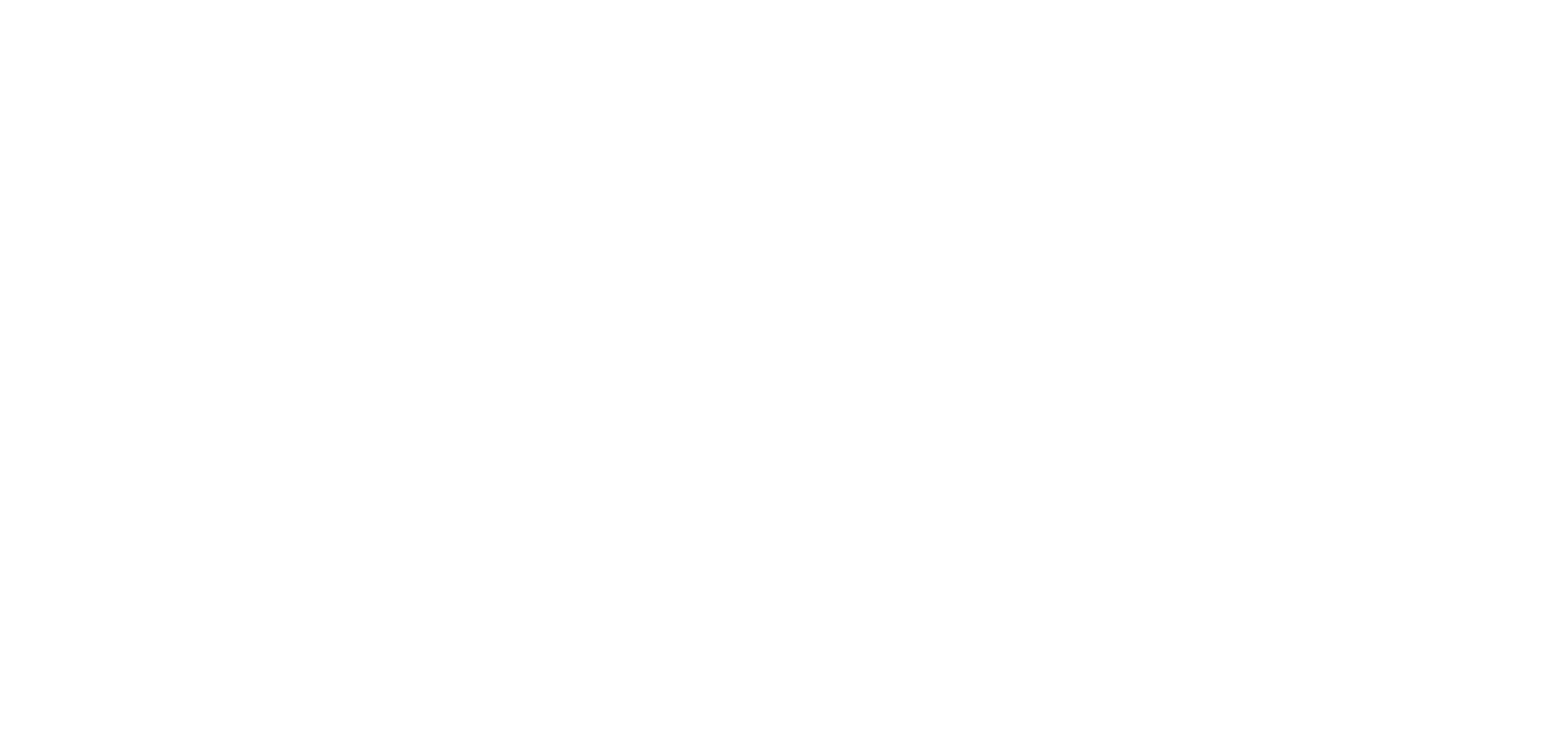
The x-T phase diagram shows different interesting features. Let’s consider pure liquids. Liquid ⁴He becomes superfluid below 2.17K, and pure ³He does not show such a phase transition in the temperature range we can consider for a dilution fridge. We note that ⁴He superfluidity terminates for ³He concentrations higher than 67.5%. At this point, the λ-line meets the phase separation line, below which a forbidden region (two-phases) exits. This means that below this line, the two isotopes are only miscible for some particular concentrations. On the side of this two-phase region, there is a light concentrated phase that is rich in ³He, and a heavy dilute phase, rich in ⁴He. The concentration of the ³He in those two phases depends upon the temperature. Because the enthalpy of ³He in the two phases is different, it is possible to cool down the mixture by evaporating the ³He from the concentrated phase into the dilute phase. We may note on the ⁴He-rich side, that the concentration of the dilute isotope does not rich zero when decreasing the temperature close to 0K. The ³He concentration rather reaches the finite concentration of 6.6%, even for T=0K. This property is of paramount importance for the design of a dilution fridge.
The following figure shows schematics of the ³He/⁴He dilution refrigerator. When the refrigerator is started, the ³He/⁴He mixture is condensed into the dilution unit. This allows the cooling down of the mixture down to 1.2K. The tricritical point is at 0.86K so it is the temperature needed to get phase separation. Hence the still is being cooled down to this point with a ⁴He flow, resulting in cooling down the incoming ³He before it enters the heat exchangers and mixing chamber. This later results in phase separation. Gradually, the rest of the dilution unit is cooled down to the point where the phase separation occurs.
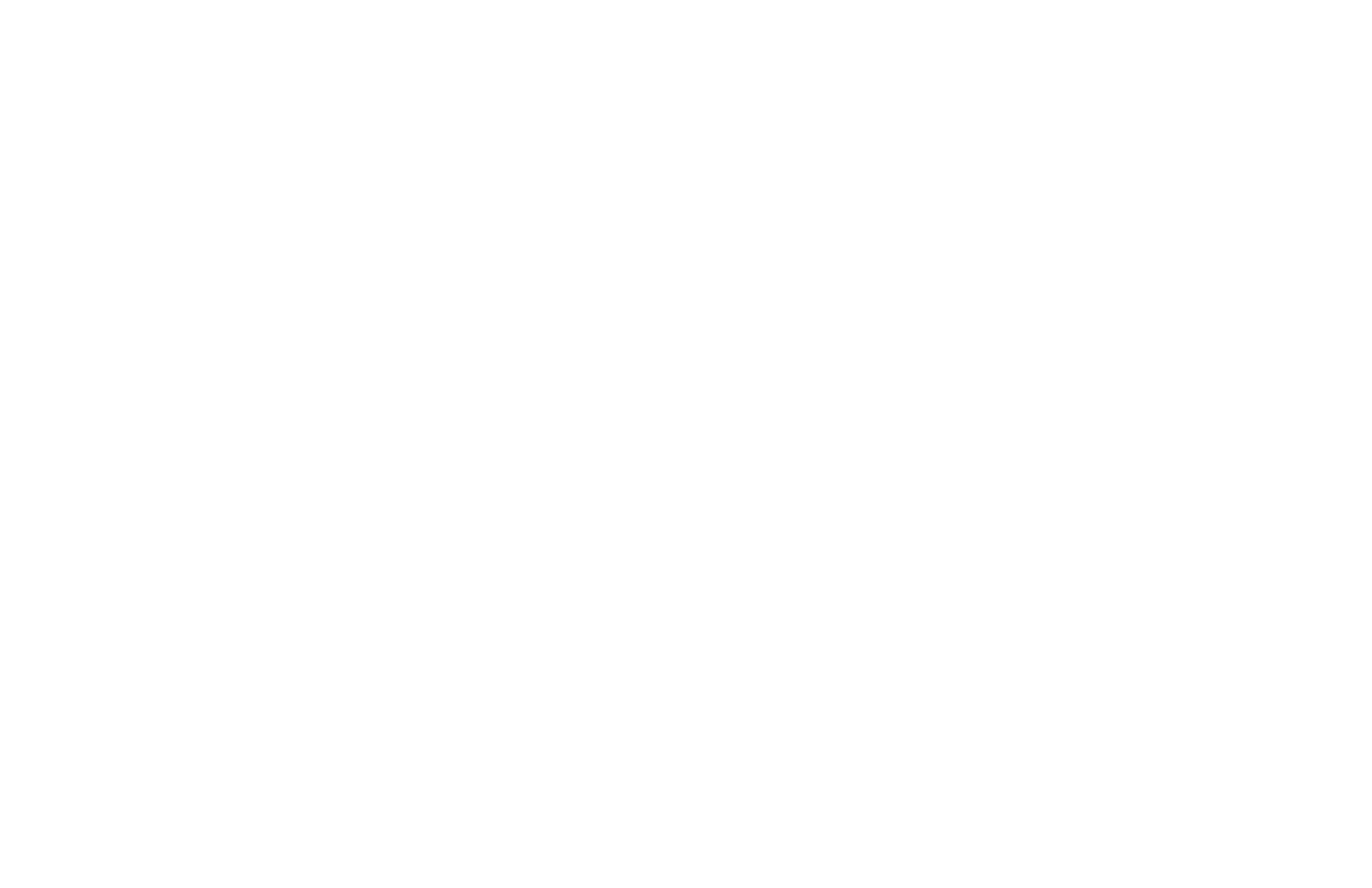
Operating Principle
In the operating system, the ³He is extracted from the dilute phase, and returned to the concentrated phase, keeping the system in a dynamic equilibrium. This is done by pumping away ³He from the liquid surface in the still, at temperature at which the pressure of ³He is an order of magnitude higher than the one of ⁴He.
As a result the ³He concentration in the dilute phase in the still becomes lower than in the still, that allows the osmotic pressure to drive a flow of ³He to the still.
Wiring and Connections
The device that needs to be cooled down is fixed over the mixing chamber, ensuring a good thermal contact with the dilute phase. All the connections to the room temperature equipment are anchored at different points of the fridge to reduce the heat load on the mixing chamber and limit heat exchanges with outside. Within the cryostat, it is important to minimise the head load. One way to conduct heat within the measured sample is through the wires that are linked to room temperature connections. For this reason, it is needed to chose materials that have a low thermal conductivity. Unfortunately, a good electrical conductivity often comes with a high thermal conductivity, except for superconducting materials that have no electrical resistance and negligible thermal conductivity. For this reason, conventional superconducting wires can be used below 8K to minimise head load on the sample stage.
Other wires can also be used. They are thermally anchored (we call this thermally sunk) to thermalised plates to ensure the wire is at the required temperature.
Limitations
There is no fundamental limitation on how low can a dilution fridge operate. However, most of the commercial refrigerators are limited above 2mK, because both the viscosity and the thermal conductivity of the mixture would increase as the temperature is lowered. To prevent this, the diameter of the tubes should be reduced and their lengths increased. This would make the volume of the fridge increase madly.
References
- Pobell F 2007 Matter and Methods at Low Temperatures (Springer)
- Hall HE, Ford PJ, and Thomson K 1966 A helium-3 dilution refrigerator Cryogenics. 6 (2): 80–88.